Source: Soraa
All of us have, at some point or another, complained about the use of lumens as a metric – for a variety of reasons. First, lumens are not very accurate as a proxy for perceived brightness. Second, there is a temptation to increase lumens by ‘cheating’, for instance on the chromaticity of a lamp – ponder this next time you are subjected to a depressingly greenish fluorescent lamp! Third, the lumen race pushes the industry to compromise on competing aspects, especially color rendition. If two LEDs emit the same number of optical watts, but one generates more long-wavelength photons to properly render deep-red tones, it is by definition penalized in terms of lumens.
A more informative metric for assessing the efficiency of an LED light source is optical watts per electrical watt, also known as the white efficiency. This physical quantity captures the efficiency by which electrical input power is converted to white optical power or white light. More importantly, the white efficiency also tells us how much of the input electrical power is lost and converted to heat (instead of light). Due to the limited heatsinking in realistic systems, this heat increases temperature, which limits reliability and thus determines the maximum drive power of the system. Today, this heat load is a key limiting factor in designing denser, brighter systems.
Therefore, although lumens per watt is central to marketing an LED lamp or fixture product, white efficiency matters much more to the engineer. An example where this nuance matters: Soraa’s LEDs are based on violet pump dies; these violet photons may be penalized in terms of lumens – but the efficiency of our violet dies is very high, meaning that emitting this violet light generates little waste heat. Thus, the cost in terms of thermal budget is in fact not problematic.
What factors cause an imperfect white efficiency? The main culprits are the efficiency of the pump LED, the phosphors’ quantum yield, the Stokes shift when converting pump light into phosphor light, and the residual optical absorption in the system. When compounding these effects, many LEDs today have a white efficiency of about 40-50%.
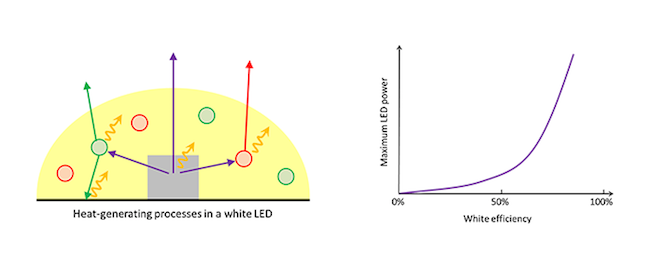 |
(Left) Heat is generated in a white phosphor-converted LED due to various factors: pump LED’s imperfect efficiency, phosphor conversion (Stokes loss and imperfect quantum yield), and optical absorption in the package. (Right) Sketch of the relationship between white efficiency and the maximum power an LED can sustain. (Soraa/LEDinside) |
Improving white efficiency is all the more crucial because it has a self-enhancing aspect: waste heat raises temperature, which degrades the efficiency of the LEDs and phosphors, leading to further wasted heat in a vicious cycle. Fortunately, the converse is true: any technological leap which increases efficiency produces a compounded improvement, because it means lower operating temperature and thus a second-order improvement on efficiency. This virtuous cycle is sizeable enough to be relevant in today’s systems. That is one reason why efficiency gains still matter: there may be fewer low-hanging fruits in terms of direct improvement than ten years ago, but each additional percent becomes vastly beneficial. Taken to an extreme, a perfectly efficient system could be driven with infinite power!
This is also why, in the long term, multi-primary systems will be an important technology: by replacing the pump LED + phosphor paradigm with a system of four or more primary LEDs combined to emit white light, extremely high white efficiencies can be envisioned. The challenge in developing some of these primary LEDs (especially at green and yellow wavelength) is of course gigantic – but that is a good problem to have for scientists!